Is Au+ Paramagnetic Or Diamagnetic
Abstract
Magnetic materials are ordinarily classified into a distinct category such as diamagnets, paramagnets or ferromagnets. The enormous progress in materials scientific discipline allows one nowadays, however, to change the magnetic nature of an element in a material. Gilded, in majority form, is traditionally a diamagnet. But in a ferromagnetic environment, it tin adopt an induced ferromagnetic moment. Moreover, the growth of gold under sure conditions may lead to a spontaneous ferromagnetic or paramagnetic response. Here, we report on paramagnetic gold in a highly matted Au–Ni–O alloy and focus on the unusual magnetic response. Such materials are mainly considered for plasmonic applications. Thin films containing Au, Ni and NiO are fabricated by co-deposition of Ni and Au in a medium vacuum of 2 × x−ii mbar. Every bit a consequence, Au is in a fully disordered land forming in some cases isolated nanocrystallites of up to 4 nm in bore as revealed by loftier resolution transmission electron microscopy. The disorder and the surround, which is rich in oxygen, lead to remarkable magnetic properties of Au: an induced ferromagnetic and a paramagnetic country. This can be proven past measuring the ten-ray magnetic circular dichroism. Our experiments evidence a way to found and monitor Au paramagnetism in alloys.
Introduction
Gold (Au) is an inert noble transition metal with 5d electrons. The crystal structure is face-centered cubic (fcc). Information technology is known as a typical diamagnetic material with negative magnetic susceptibility. Every bit an isolated cantlet, Au has completely filled 5d states, and then there is no magnetic moment. In metal form, at that place is a pocket-sized number of holes in the vd band due to cocky-hybridization effects. During the last 15 years, outstanding scientific research on Au has been performed using element-specific techniques carried out in synchrotron-radiations facilities. It has been demonstrated by ten-ray magnetic circular dichroism (XMCD) that Au tin can larn an induced magnetic moment when it forms alloys or layered-motion-picture show structures with 3d ferromagnetic transition metals1,ii,3. Ferromagnetism in tiny Au nanoparticles, without or with thiol-group capping, has also been reported4,v,6. More recently, paramagnetism in both, bulk Au and Au nanoparticles has attracted the scientific involvement: Suzuki et al. probed Pauli and orbital paramagnetic state in bulk golden by XMCD7. Bartolomé et al. showed that for Au nanoparticles deposited on a Sulfolobus acidocaldarius S layer, the magnetic moment per paramagnetic Au atom was 25 times larger than in other Au nanoparticles measured at that time8. From these studies, an additional interest emerged for further investigation of Au paramagnetism on dissimilar probe systems and in various environments.
According to ref.8, the key factor for discovering paramagnetic gilt is the increase in the number of vd holes. This may originate from a accuse transfer from Au atoms in nanoparticles to other atoms and/or the existence of Au in the form of tiny crystals or clusters with open bonds. Therefore, in this work, nosotros intentionally fabricated a highly disordered system containing tiny nanoparticles of Au and Ni. A big percent of Ni is oxidized. By ways of XMCD experiments, we record for Au an induced ferromagnetic state for minor magnetic fields followed past a paramagnetic state up to 17 T. The two contributions tin be separated. Our experiments prove a style to establish and probe Au paramagnetism in alloys. Upward to now, merely one paper reported on Au paramagnetism, exhibited by nanoclusters grown on an archaeal-cell-wall surface layer8. Our piece of work shows a more systematic way to grow nano-systems in which it is possible to detect Au paramagnetism. Terminal only non least, Au in NiO is an interesting, plasmonic system9,x,11. It finds many applications, amongst others, in catalysis, photovoltaics and gas sensing.
Results
In Fig. 1, a cross-section scanning manual electron microscopy (Stalk) epitome and the results of energy-dispersive Ten-ray spectroscopy (EDS) analysis are shown. The chemical analysis presented past EDS-STEM images indicates that Au atoms are homogeneously distributed in the Au-Ni-O thin motion picture. Within the accuracy of the EDS detector of v%, the film contains about 8 at. % Au, with well-nigh ii thirds of Ni being oxidized.
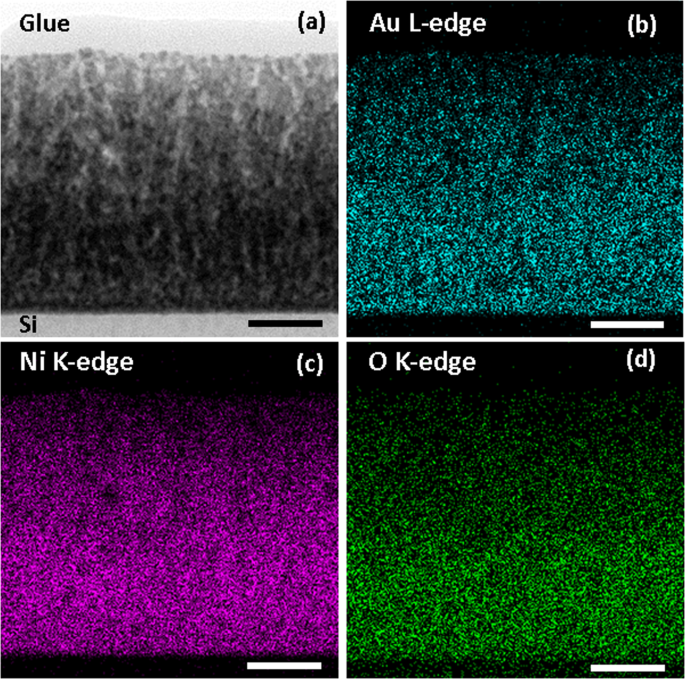
(a) Annular bright-field STEM image of Au-Ni-O thin motion picture, (b–d) corresponding EDS-Stalk elemental mapping of the gold, nickel and oxygen elements, respectively. The scale bar is thirty nm.
In Fig. 2(a), a cross-section high-resolution transmission electron microscopy (HRTEM) paradigm is shown. We clearly see the polycrystalline nature of the Au-Ni-O sparse motion-picture show. The average size of the crystallites is less than 5 nm in diameter. They feature various shapes. The overall thickness of the film, determined with high precision, was 103 ± 1 nm. Electron diffraction [Fig. two(d)] confirms the presence of both, NiO and Ni Fm-3m phases. The presence of Au rings could not be verified in the electron diffraction of large areas of the image due to fact that the Au interplanar distances are very close to the NiO ones. Furthermore, taking into account the enlargement of the NiO rings due to the small size of the crystallites, they most probably overlap with the ones from Au.
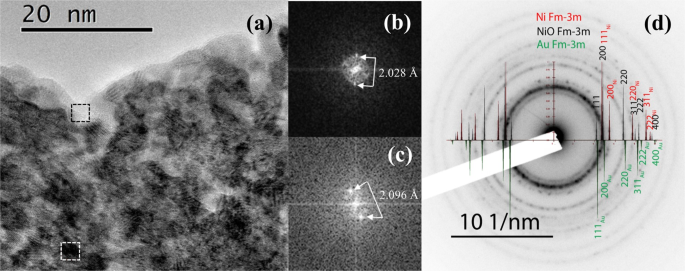
(a) HRTEM epitome of Au-Ni-O thin film close to the surface of the 100 nm flick, (b,c) FFT of the square areas noted in (a). Interplanar distances extracted from black areas stand for to Au and from light-color areas to NiO. (d) Electron-diffraction pattern of the 100 nm Au-Ni-O film.
Since nosotros could not carve up NiO from Au contributions by electron diffraction, a thin TEM lamella has been prepared. To enhance the contrast, a series of under-focus HRTEM images close to the thinnest expanse of the TEM lamella has been taken in guild to measure direct the interplanar distances. From fast Fourier transform (FFT), extracted from small blackness and small bright areas in Fig. 2(a), the measured interplanar distances were found to correspond to Au [Fig. 2(b)] and to NiO [Fig. 2(c)], respectively. For the majority of grey areas, the size of the Au crystals is smaller than the thickness of the TEM lamella and, as a effect, the measured distance is averaged between Au and NiO. Note also that HRTEM in a like but merely 10 nm thick film showed the presence of partially amorphous material; simply part of Au was in crystalline form with a few isolated grains up to 2 nm in diametereleven. In the nowadays work, we could not identify amorphous material, so Au is in cubic fcc crystalline form consisting of tiny nanocrystals (smaller than almost 4 nm, meet Fig. 2(a), blackness areas). The existence of open bonds (Au in form of incomplete crystals or clusters) cannot be ruled out either. In ref.11, a function of Ni was in the hexagonal hcp stage. Hexagonal Ni in bulk form does not touch on the magnetic backdrop of its environment as information technology is non-magnetic12,13. In the form of modest nanoparticles, hcp Ni could also show some antiferromagnetismfourteen. However, in the thick Au-Ni-O film of this written report no hcp Ni was evidenced. The common conclusion of both studies, this 1 and ref.eleven, is that they bear witness evidence of a highly disordered arrangement.
In Fig. 3, the spectra of Ten-ray absorption spectroscopy (XAS) and XMCD at the Ni K-border of our film are plotted. The XAS recorded on the film is a linear combination of XAS of Ni in NiO and pure Ni. According to EDS, the total corporeality of Ni in the sample is 55%, of which 35% is NiO and 20% is Ni metal. The XAS measures all Ni atoms of the sample, i.e. the spectral shape of the XAS is a mixture betwixt NiO and Ni metallic. The XAS spectrum resembles more the one of NiO (crystallized in a slightly rhombohedrally-distorted cubic NaCl structure)15 than the one of majority fcc Ni16,17. This observation confirms the results of EDS and HRTEM that most of Ni is oxidized. On the other manus, the spectral shape of the XMCD bespeak corresponds for the most office to the ane of Ni metal. However, this XMCD point is much smaller than the one expected for fcc Ni. This can be conspicuously seen by a direct comparison of the XMCD Ni indicate of Fig. 3 to the 1 actualization in ref.17 for pure fcc bulk Ni. Since NiO is an antiferromagnet, it has zero contribution to the XMCD indicate. Hence, the modest Ni M-edge XMCD is due to the pocket-size per centum of fcc Ni in the sample. From its aamplitude, we can deduce that at most 20% of the Ni atoms belong to ferromagnetic Ni metallic. Differences in the line shape of the XMCD as compared to bulk Ni17 may arise from the modest size effects (low dimensionality) of the Ni fcc clusters. These furnishings may modify the density of states and, consequently, the orbital magnetism, which is probed through the M-edge XMCD signal. A farther reduction of the signal may originate from disorder. Indeed, disorder was shown to reduce Ni 1000-edge XMCD in NiMnGa alloys18. Finally, one has to consider that in some cases disorder and strain may induce a ferromagnetic phase in NiO, see eastward.m. ref.19 and references therein. In this case, the average magnetization increases with decreasing particle size, which is correlated to an increase in the number of uncompensated spins at the surface with respect to the core. Such a phase could as well contribute to the Ni XMCD signal.
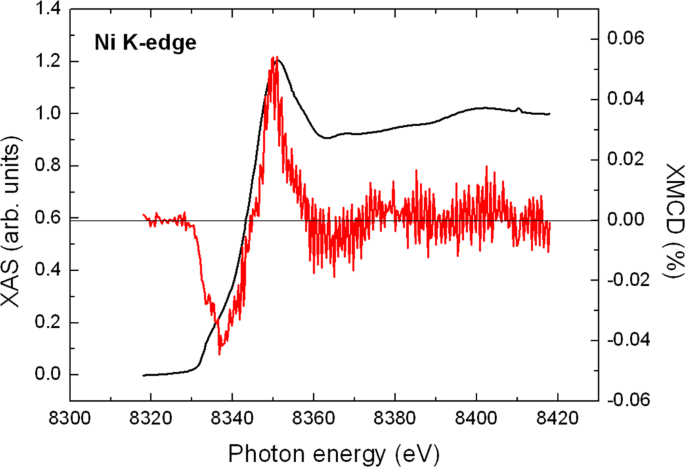
XAS (smoothen line) and XMCD (noisy line) recorded at the 1000 edge of Ni in a matted Au-Ni-O film. The XAS spectrum is normalized to unity.
In Fig. 4, the XAS and XMCD spectra recorded at 295 K under a field of 17 T at the Fifty 3 and L 2 edge of Au are plotted for the Au-Ni-O flick. For the XAS spectra, the ratio of the L three and Fifty 2 intensity was normalized to 2.24:1 according to ref.xx. This value is also very close to the value of 2.two:one reported past Tyson et al.21. The finite XMCD betoken reveals that Au possesses a magnetic moment. Knowing the direction of the magnetic field and the helicity of the beam, we conclude that Au is polarized parallel to the magnetic field and to the Ni magnetic moment.
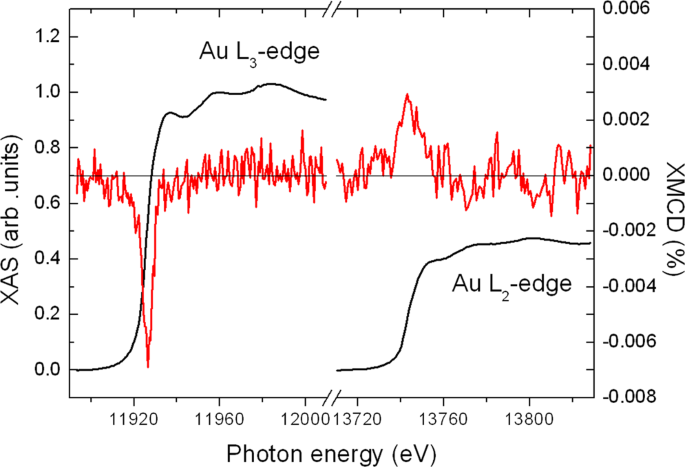
XAS (smooth line) and XMCD (noisy line) recorded at the L 3 and L 2 edge of Au in a disordered Au-Ni-O film. At the L iii edge, the XAS spectrum is normalized to unity.
By setting the monochromator at the maximum of the 50 3 XMCD signal of Au and increasing the magnetic field to 17 T, we take recorded an element-specific magnetization bend for Au. It is a well-known advantage of the XMCD technique to let recording of element-specific magnetization curves22,23. The outcome is plotted in Fig. 5. A rather precipitous increase is seen at applied fields lower than 1 T, followed past a slow linear response up to 17 T. Since the field increment is 1 T, the behavior nigh zilch is not known in particular. Still, the low-field beliefs may be attributed to an induced magnetic moment of Au that is spin-polarized past ferromagnetic Ni, Alternatively, it might originate from a polarization of Au by superparamagnetic Ni. To analyze this, magnetization measurements were performed with a Quantum Design superconducting quantum-interference device vibrating-sample magnetometer (SQUID VSM). As illustrated in Fig. six, a hysteresis loop of the 200 nm thick sample has been recorded at 300 Grand. The corrected magnetization loop, i.eastward., afterward subtraction of a contribution of the diamagnetic Si substrate, is typical for a soft ferromagnetic polycrystalline material with a coercivity of 7.5 mT. The hysteresis is completed at about 0.5 T and the magnetization reaches a saturation value of 2 × x−6 emu.
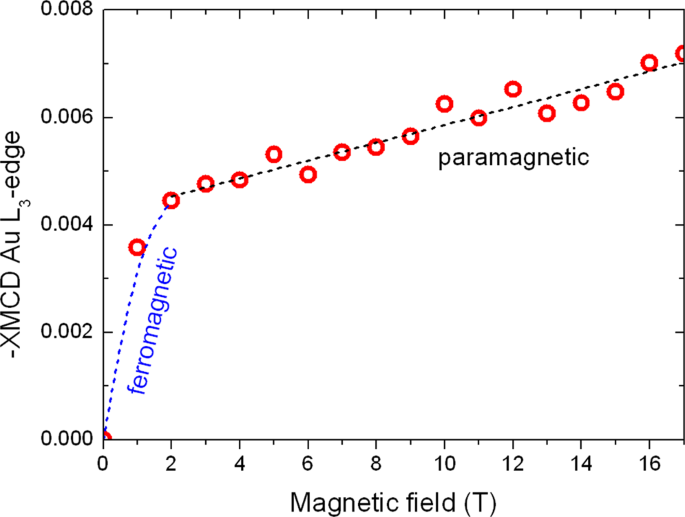
Magnetization curve recorded at the L 3 border of Au in a disordered Au-Ni-O movie. The curve shows (below 1 T) an induced ferromagnetic and (above 1 T) a paramagnetic behavior.
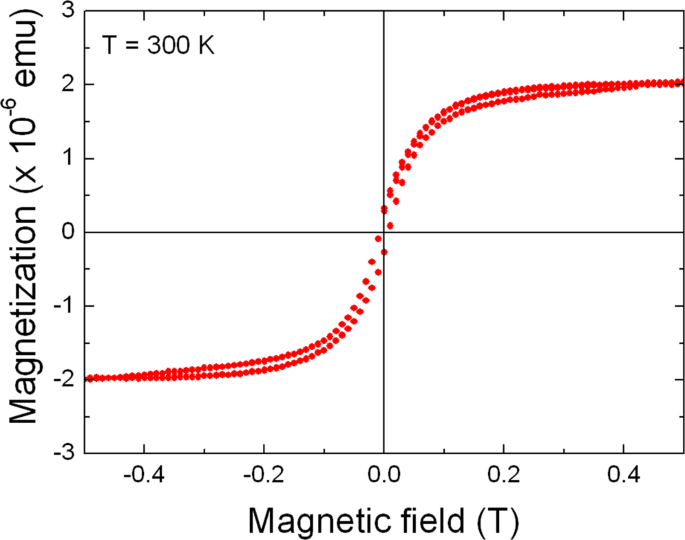
SQUID VSM hysteresis loop recorded with the magnetic field applied parallel to the film surface.
Discussion
The induced magnetic moments of Pd, Pt, and even Au, in a ferromagnetic environment, probed by XMCD, was the target of a few papers in the nineties and later. For the first 2 elements, there were results for magnetic polarization by Fe, Co and Ni24,25. Au, equally far as we know, is known to exist polarized only by Co1 or Feiii. Therefore, it is reasonable to expect that Ni could also spin-polarize Au, e.g. in ferromagnetic Ni/Au multilayers or NiAu alloys. This is the beliefs of Au in our sample under fields upwardly to approximately 1 T, as i tin can conclude from Figs 5 and 6. Note that NiO is an antiferromagnet and such transition-metal oxides were, upwardly to at present, not shown to exist able to produce proximity effects even to easily spin-polarizable elements such as Pt26,27.
Even more interesting is the observation of the deadening linear increase, which is typical for paramagnetic materials. Au is not a paramagnet merely it has been shown to possess a very small Pauli paramagnetic susceptibility in bulkseven. Under special circumstances, in the grade of nanoparticles grown on special substrates, information technology can show a stiff paramagnetic behavior8. In guild to quantify the paramagnetic beliefs of Au in our system, the susceptibility of Au has to be adamant, which is defined equally χ = dM/dH, where K is the magnetization and H the magnetic-field force. Therefore, we applied on the spectra of Fig. iv the analysis process for 4d and vd elements with small-scale white-line intensities, adult for Pd and Ag in Fe/Pd multilayers24. The XAS spectra at the 50 edges of the transition metal in the film has to exist compared with bulk Ag or Au textile (usually a pure metallic gold foil is measured as a reference) and the number due north h of d holes of Ag or Au has to be taken into business relationship24,25. This method works well if the sample has the fcc structure of the reference.
In Fig. 7, we plot the XAS spectra at the L 3 edge of Au in our pic, in a fcc Co12/Au4 multilayerone (the indices 12 and 4 refer to the number of diminutive layers in a multilayer menstruum), in a fcc Au50Fe50 blendthree and in a fcc reference gilt foil. The reference-foil measurements have been done using a 0.1 mm thick gold foil from Goodfellow with 99.999 at.% purity, which gave the same results as a Au single crystal (99.999 at.% purity, from MaTeck). The free energy centrality of the spectrum of Au in the reference foil was stretched in gild to lucifer the one of Au in the blend, where the atomic distances are unlike from bulk Au23. One may observe that (i) the spectroscopic features of Au after the border are quite similar for the latter three fcc samples but less for Au in Au-Ni-O. The simple, almost sinusoidal spectroscopic profile of Au in our Au-Ni-O sample reminds of the one of elements in amorphous environments28. In our picture show, we suggest that this is due to big disorder. (ii) In the case of Co/Au multilayers, at that place is practically no alter of the white-line intensity of Au as compared to the reference Au foil. However, white-line intensity increases for both, Au in the AuFe alloy and Au in our sample. In AuFe alloys, this outcome was attributed to hybridization between Au 5d and Fe iiid states, which resulted in a charge transfer from Au to Atomic number 26iii. Obviously, between the less-reactive Co and Au no such charge transfer occurs, leaving the white-line intensity of Au unaltered. One should also expect that the presence of the almost inert Ni would have a similar negligible influence on the white-line intensity of Au in the Au-Ni-O motion-picture show. Therefore, the white-line intensity increment could exist attributed to a likely charge transfer from Au to oxygen or NiO. Indeed, XAS experiments in Au2O3 revealed a very big increase in the white line at the L iii absorption edge of Au29. On the other hand, a less significant white-line intensity increase, more or less similar to our case, was reported for Au nanoparticles about 10 nm in diameter embedded in a NiO matrix with incorporated hydrogenfifteen. Combination of XAS with measurements of the electrochromic issue revealed a charge transfer betwixt NiO and Au15. Too this accuse transfer, the size of Au clusters, e.g. in a matted material, can also exist idea responsible for white-line intensity changes30. Therefore, both effects could contribute to the white-line increase observed in our moving picture.
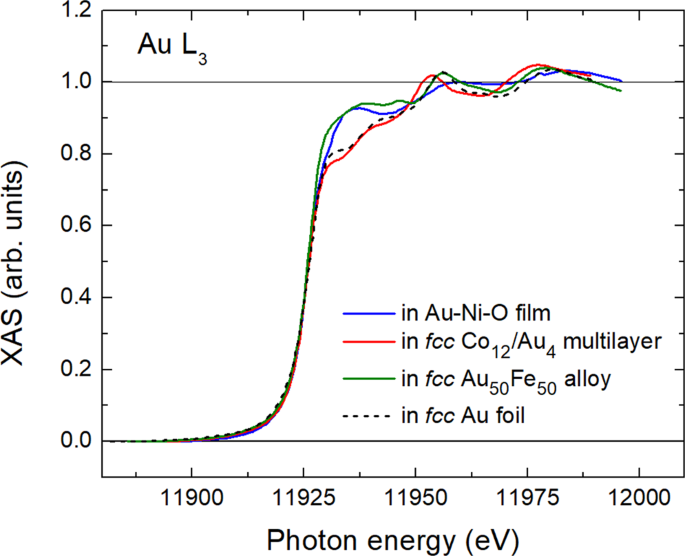
Normalized XAS spectra recorded at the Fifty 3 border of Au in various specimens equally indicated.
The fact that the XAS of Au in our sample does not match the one of fcc Au, because the lattice symmetry is dissimilar, does not allow analyzing our spectra following ref.26. In such a case, an guess method tin can be followed, adopted for hcp CoCrPt alloys31. It has too been used in the evaluation of the Au moment in a bcc Au97Fe3 alloy3. After normalizing the XAS spectra, nosotros compare the integrated XMCD spectra of Au in our movie to the ones of Au in the pure metallic fcc gold foil. This idea is justified by the fundamental principle that the sum rules establish a unproblematic linear relationship betwixt the magnitude of the XMCD signal and the magnetic moment, for a detailed discussion, encounter ref.32. Application of this principle to the spectra of Au in Fig. 4 allows one to gauge with an accuracy of the order of 20% a very small total vd magnetic moment of about 0.016 μB/cantlet for Au in our film in a field of 17 T. This value is more than 1 order of magnitude smaller than the 5d moment of Au in a Au50Fe50 blend. As Fig. 5 shows, practically sixty% of this value is due to induced ferromagnetic moment and the residue is a paramagnetic contribution.
By scaling the y axis of Fig. 5 in μB/at of Au, one may gauge from the slope of the linear role of the curve the paramagnetic susceptibility of Au. The value nosotros calculate is χ = 3.5 × 10−seven μB/at-Oe. In SI units, this amounts to χ ~ ii × 10−three. This value falls well within the range of susceptibility values observed in paramagnetic materials33. The value is comparable to the susceptibility at 10 K of Au nanoparticles deposited on an archaeal-cell-wall surface layerviii. The charge transfer in that case was constitute to be 0.152 electrons8. In our work, nosotros can requite just a rough estimate because the white-line intensity in our sample is comparable to the one in a AuFe blend (Fig. 7). Thus, the charge transfer is about 0.045 electrons. This value may exist adamant from the Au spectra of ref.3 and the use of a Au spectra for an AuivMn alloy, in a similar manner as described in ref.8.
An interesting point to be discussed is the key origin of the paramagnetic Au behavior. The nearly straightforward justification would be the existence of localized moments on the tiny Au nanocrystallites and clusters. In principle, Pauli paramagnetism could accept been expected past Au crystallites that are substantially larger than ours, in order that proper electronic bands of Au tin can develop. Pauli paramagnetism would probably upshot in smaller susceptibility values6 than those determined in this piece of work. Nevertheless, a few papers suggest that the metal character is preserved even for pocket-size clusters of gold34, sodium35 and palladium36. In the case of Pd, a big susceptibility was observed at low temperatures, while Pauli paramagnetism was noticeable even at room temperature. Therefore, in the present work, our experiments cannot conspicuously exclude the being of Pauli paramagnetism.
Conclusions
In this work, strongly disordered films of Au-Ni-O have been grown. The Au concentration was almost 8 at.%. Via XMCD measurements, we observed an induced magnetic moment of Au, showing as a function of applied magnetic field an initially precipitous increase, followed past a slow increase upward to 17 T revealing a paramagnetic contribution. The total magnetic moment of Au at 17 T is 0.016 ± 0.003 μB/atom; 60% of information technology is due to a ferromagnetic contribution and the balance is attributed to Au paramagnetism most probably due to localized moments. A paramagnetic susceptibility χ ~ 2 × x−three (in SI units) is constitute for Au; this value falls well inside the range of susceptibility values observed in paramagnetic materials. Finally, we have demonstrated that it may not be extraordinary to observe paramagnetic gold in nature. The key idea is to put Au atoms in a disordered land in tiny isolated crystallites (with diameter smaller than nigh 4 nm as the nowadays works shows) or in clusters surrounded by atoms that may attract some accuse from Au, such as oxygen atoms. Our work could open the route for the training of samples with similar methodologies in gild to make further investigations on the interesting and up to now rare phenomenon of Au paramagnetism.
Methods
Two films, 100 nm and 200 nm thick, were prepared nether identical experimental conditions in medium vacuum with base of operations pressure level 2 × 10−2 mbar by co-degradation of Au and Ni using direct-current magnetron sputtering. The medium vacuum results in the formation of a precursor-fabric sparse moving picture where a significant amount of oxygen is trapped inside the movie. The presence of oxygen and the relatively low deposition temperature, which is room temperature, result in a quenching of the deposited material prohibiting crystal growth. This is obvious for room-temperature growth due to low improvidence rates. The presence of oxygen also results in a decreased size of crystallites. As for most metals during thin-film growth, oxygen is get-go incorporated in the grain boundaries prohibiting crystal growth, see e.g. ref.37. Therefore, every bit is shown past HRTEM, the thin film consists of tiny nanocrystals while part of it is amorphous. This material was previously shown to produce after annealing high-quality plasmonic sparse films11.
Annular bright field imaging and EDS were nerveless by STEM with a JEOL 2100 F FEG microscope operating at 200 kV with 0.2 nm resolution in scanning way. EDS experiments were carried out with the novel Centurio silicon drift detector with a large solid angle of upward to 0.98 steradians from JEOL. HRTEM experiments have been performed in a JEOL 2011 microscope with a point resolution of 0.19 nm. All TEM lamella have been prepared using Multiprep semi-automatic tripode polishing and finished with Ar-ion thinning.
The XMCD experiments were performed at the ID12 beamline of the European Synchrotron Radiation Facility in Grenoble (France) at the L 3 and Fifty two edge of Au at 295 G and the K edge of Ni at 2 Yard using highly efficient fluorescence-yield detection mode in backscattering geometry38. XAS spectra were recorded under external fields upwardly to 17 T. The XAS spectra at the Ni K edge and Au L 3 edge were normalized to unity. The experiments were performed at grazing incidence (fifteen° with respect to the pic plane). The progress in 3rd-generation synchrotron-radiation facilities has made possible the detection of the Au XMCD signal at the Au 50 edges with relatively good signal-to-noise ratio for a 100 nm thin film containing just about 8 at. % Au. To exclude any experimental artifacts, the XMCD spectra were recorded either by changing the helicity of the incoming calorie-free or by inverting the direction of the external magnetic field.
Finally, magnetic measurements were realized on a 3.5 × 4 mm2 slice of a 200 nm thick film with the external magnetic field applied parallel to the flick surface at 300 Thou. These measurements are based on the extraction technique, nether magnetic fields up to 5 Tesla, using a SQUID VSM. The diamagnetic betoken originating from the silicon substrate was subtracted from the raw measurements.
References
-
Wilhelm, F. et al. Magnetic moment of Au at Au/Co interfaces: A direct experimental determination. Phys. Rev. B 69, 220404(R) (2004).
-
Bartolomé, J. et al. Magnetic polarization of noble metals by Co nanoparticles in 1000-capped granular multilayers (M = Cu, Ag, and Au): An x-ray magnetic circular dichroism written report. Phys. Rev. B 77, 184420 (2008).
-
Wilhelm, F. et al. Au and Fe magnetic moments in matted Au-Fe alloys. Phys. Rev. B 77, 224414 (2008).
-
Crespo, P. et al. Permanent Magnetism, Magnetic Anisotropy, and Hysteresis of Thiol-Capped Gold Nanoparticles. Phys. Rev. Lett. 93, 87204 (2004).
-
Yamamoto, Y. et al. Direct Observation of Ferromagnetic Spin Polarization in Gold Nanoparticles. Phys. Rev. Lett. 93, 116801 (2004).
-
Garitaonandia, J. S. et al. Chemically Induced Permanent Magnetism in Au, Ag, and Cu Nanoparticles: Localization of the Magnetism by Chemical element Selective Techniques. Nano Lett. 2, 661–667 (2008).
-
Suzuki, Thousand. et al. Measurement of a Pauli and Orbital Paramagnetic State in Majority Gold Using X-Ray Magnetic Circular Dichroism Spectroscopy. Phys. Rev. Lett. 108, 047201 (2012).
-
Bartolomé, J. et al. A. Strong Paramagnetism of Gold Nanoparticles Deposited on a Sulfolobus acidocaldarius S Layer. Phys. Rev. Lett. 109, 247203 (2012).
-
Ando, M., Kobayashi, T. & Haruta, One thousand. Combined effects of modest gold particles on the optical gas sensing by transition metallic oxide films. Catal. Today 36, 135–141 (1997).
-
Ohodnicki, P. R. Jr. et al. High temperature optical sensing of gas and temperature using Au-nanoparticle incorporated oxides. Sens. Human action. B 202, 489–499 (2014).
-
Grammatikopoulos, S. et al. Growth of Au Nanoparticles in NiO via Brusk Annealing of Precursor Material Thin Movie and Optimization of Plasmonics. Phys. Status Solidi A 214, 1700303 (2017).
-
Poulopoulos, P., Kapaklis, Five., Politis, C., Scweiss, P. & Fuchs, D. Non-magnetic hexagonal nanocrystalline ni films grown by radio frequency magnetron sputtering. J. Nanosci. Nanotechnol. 6, 3867–70 (2006).
-
Kapaklis, 5. et al. Structure and magnetic backdrop of hcp and fcc nanocrystalline thin Ni films and nanoparticles produced by radio frequency magnetron sputtering. J. Nanosci. Nanotechnol. x, 6024 (2010).
-
Kleibert, A. et al. Direct ascertainment of enhanced magnetism in individual size- and shape-selected 3d transition element nanoparticles. Phys. Rev. B 95, 195404 (2017).
-
Ferreira, F. F. & Avendano, E. Reversible Electronic Charge Transfer between Au Nanoparticles and Electrochromic NiO Matrices upon Electrochemical Cycling. J. Phys. Chem. C 111, 16608–16612 (2007).
-
Bouldi, N. et al. X-ray magnetic and natural round dichroism from get-go principles: Adding of K- and L1-edge spectra. Phys. Rev. B 96, 085123 (2017).
-
Torchio, R. et al. X-Ray Magnetic Circular Dichroism Measurements in Ni up to 200 GPa: Resistant Ferromagnetism. Phys. Rev. Lett. 107, 237202 (2011).
-
Chaboy, J. et al. XAS and XMCD report of the influence of annealing on the diminutive ordering and magnetism in an NiMnGa blend. J. Phys.: Condens. Affair 21, 016002 (2009).
-
Ravikumar, P., Kisan, B. & Perumal, A. Enhanced room temperature ferromagnetism in antiferromagnetic NiO nanoparticles. AIP Advances 5, 087116 (2015).
-
http://www-cxro.lbl.gov/optical_constants.
-
Tyson, C. C. et al. Accuse redistribution in Au-Ag alloys from a local perspective. Phys. Rev. B 45, 8924 (1992).
-
Goering, E., Fuss, A., Weber, W., Will, J. & Schütz, Grand. Element specific x-ray magnetic circular dichroism magnetization curves using total electron yield. J. Appl. Phys. 88, 5920 (2000).
-
Radu, F., Abrudan, R., Radu, I., Schmitz, D. & Zabel, H. Perpendicular exchange bias in ferrimagnetic spin valves. Nature Commun. 3, 715 (2012).
-
Vogel, J. et al. Structure and magnetism of Pd in Pd/Fe multilayers studied by x-ray magnetic circular dichroism at the Pd L2,3 sedges. Phys. Rev. B 55, 3663 (1997).
-
Poulopoulos, P. et al. X-ray magnetic round dichroic magnetometry on Ni/Pt multilayers. J. Appl. Phys. 89, 3874 (2001).
-
Geprägs, S. et al. Investigation of induced Pt magnetic polarization in Pt/Y3Fe5O12 bilayers. Appl. Phys. Lett. 101, 262407 (2012).
-
Collet, Yard. et al. Investigating magnetic proximity furnishings at ferrite/Pt interfaces. Appl. Phys. Lett. 111, 202401 (2017).
-
Kapaklis, V. et al. Near-edge 10-ray assimilation fine-structure fingerprints of majority-baggy and nanostructured Pd-based alloys. J. Appl. Phys. 98, 044319 (2005).
-
Weiher, N., Willneff, East. A., Figulla-Kroschel, C., Jansen, M. & Schroeder, S. L. 1000. Extended 10-ray assimilation fine-structure (EXAFS) of a circuitous oxide construction: a full multiple scattering analysis of the Au L3-edge EXAFS of Au2O3. Solid State Commun. 125, 317–322 (2003).
-
Bazin, D., Sayers, D., Rehr, J. J. & Mottet, J. J. Numerical Simulation of the Platinum LIII Border White Line Relative to Nanometer Scale Clusters. J. Phys. Chem. B 101, 5332–5336 (1997).
-
Poulopoulos, P. X-Ray magnetic circular dichroism on Pt 50-edges in Co-based materials. Int. J. Mod. Phys. B 19, 4517–4523 (2005).
-
Rogalev, A., Wilhelm, F., Jaouen, Northward., Goulon, J. & Kappler, J.-P. X-ray Magnetic Circular Dichroism: Historical Perspective and Contempo Highlights. In Magnetism: Synchrotron Radiations Arroyo ed. past Beaurepaire, Due east., Bulou, H., Scheurer, F. & Kappler, J.-P. Lecture Notes in Physics, Springer-Verlag, 697, 71–93 (2006).
-
Callister, Westward. D. Jr. Materials Science and Engineering science: An Introduction, (ed. John Wiley & Sons, Inc.) 5th edition, affiliate eighteen (New York, 2000).
-
Issendorff, B. The electronic structure of Alkali and Noble Metallic Clusters, in Handbook of Nanophysics half dozen, Sattler, K. (ed.), CRC-Press, (Boca Raton, 2011).
-
Bowlan, J., Liang, A. & de Heer, Westward. A. How Metal are Small Sodium Clusters? Phys. Rev. Lett. 106, 043401 (2011).
-
Volokitin, Y. et al. Quantum-size effects in the thermodynamic backdrop of metallic nanoparticles. Nature 384, 621–623 (1996).
-
Petrov, I., Barna, P. B., Hultman, L. & Greene, J. Eastward. Microstructural development during movie growth. J. Vac. Sci. Technol. A 21, S117 (2003).
-
Rogalev, A., Goulon, J., Goulon-Ginet, C. & Malgrange, C. Instrumentation developments for polarization dependent x-ray spectroscopies. in Magnetism and Synchrotron Radiations ed. by Beaurepaire, Eastward., Scheurer, F., Krill, Thousand. & Kappler, J.-P., Lecture Notes in Physics, Springer-Verlag 565, sixty–86 (2001).
Acknowledgements
Nosotros thank the ESRF crew for the excellent operational conditions. Nosotros thank Dr. A. Delimitis for the images of Ref. [eleven] which were helpful for this work, too. A.S acknowledges fiscal support for this projection past the FUB. The nowadays work was likewise financially supported past the "Andreas Mentzelopoulos Scholarships for the Academy of Patras". This work was partially co-financed past the European Marriage and Greek national funds through the Operational Program Competitiveness, Entrepreneurship and Innovation, under the call Inquiry – CREATE – INNOVATE (project code:T1EDK-04659). Electron microscopy was performed at the CMTC characterization platform of Grenoble INP supported past the Centre of Excellence of Multifunctional Architectured Materials 'CEMAM' No. ANR-10-LABX-44-01 funded past the "Investments for the Futurity" Program.
Writer information
Authors and Affiliations
Contributions
A.S. and A.G. participated in the XMCD beam time. P.P. and P.F. led the project. P.P., F.W. and P.F. did the main writing of the newspaper. A.Southward. and Due south.Grand. did the sample growth. Due east.S. and L.R. performed the Electron Microscopy experiments. C.O. performed the SQUID VSM measurements. F.W. and A.R. are axle line scientists at ID12, ESRF and participated in the XMCD beam time. All authors carried out information assay, participated in discussion of the results and commented on the manuscript.
Corresponding author
Ideals declarations
Competing Interests
The authors declare no competing interests.
Boosted data
Publisher's note: Springer Nature remains neutral with regard to jurisdictional claims in published maps and institutional affiliations.
Rights and permissions
Open up Access This article is licensed nether a Creative Eatables Attribution 4.0 International License, which permits use, sharing, accommodation, distribution and reproduction in any medium or format, as long as you give appropriate credit to the original author(south) and the source, provide a link to the Creative Commons license, and betoken if changes were made. The images or other third political party cloth in this article are included in the article's Creative Commons license, unless indicated otherwise in a credit line to the material. If textile is not included in the article'southward Creative Commons license and your intended employ is not permitted past statutory regulation or exceeds the permitted use, yous volition need to obtain permission directly from the copyright holder. To view a copy of this license, visit http://creativecommons.org/licenses/by/4.0/.
Reprints and Permissions
Well-nigh this commodity
Cite this article
Stamatelatos, A., Poulopoulos, P., Goschew, A. et al. Paramagnetic gold in a highly disordered Au-Ni-O alloy. Sci Rep 9, 13137 (2019). https://doi.org/10.1038/s41598-019-49457-vii
-
Received:
-
Accepted:
-
Published:
-
DOI : https://doi.org/10.1038/s41598-019-49457-vii
Comments
By submitting a comment y'all agree to abide by our Terms and Customs Guidelines. If you find something calumniating or that does not comply with our terms or guidelines please flag information technology as inappropriate.
Is Au+ Paramagnetic Or Diamagnetic,
Source: https://www.nature.com/articles/s41598-019-49457-7
Posted by: newmanmunly1988.blogspot.com
0 Response to "Is Au+ Paramagnetic Or Diamagnetic"
Post a Comment